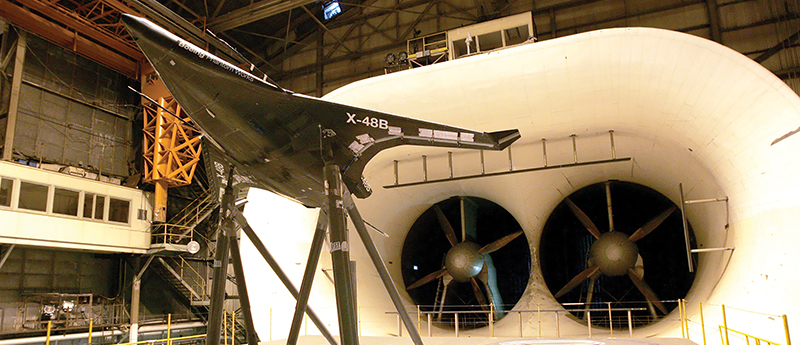
Unique Sensors Will Improve Aerodynamic Design, Aircraft Performance
NASA Technology
Even after almost 150 years of wind tunnel testing, no commercial product had ever been able to directly measure the localized force that passing air exerts on the surface of a vehicle model—until now. Known in the industry as wall shear stress, or skin friction, the force is a major factor in a vehicle’s drag, affecting its fuel efficiency and aerodynamic performance.
Thanks to two decades of research and engineering, Interdisciplinary Consulting Corporation (IC2) now has sensors that introduce the unprecedented capability to sense these forces directly.
Without this ability, engineers doing aerodynamic testing have come up with ingenious techniques like observing the change in voltage required to maintain the temperature of a heated film on a model’s surface or tracking the localized thickness of an oil film and then backing out the data to arrive at an estimate of shear stress.
“All of these methods have limitations which lead to large uncertainties, and direct measurement is the only way we’re going to get what we need,” says Cathy McGinley, head of the Flow Physics and Control Branch at NASA’s Langley Research Center.
In the mid-2000s, three University of Florida professors set out to address the problem with funding from a three-year NASA Research Announcement and an idea for a microelectromechanical systems (MEMS) sensor that could directly measure shear stress. One of those professors, Mark Sheplak, had co-founded Gainesville, Florida-based IC2 in 2001 to commercialize technology developed in his lab at the university, known as the Interdisciplinary Microsystems Group.
It took more than another decade—and considerably more NASA funding—to arrive at a product.
“For the first 10 years, the company focused on small consulting jobs and the occasional SBIR,” says company President Steve Horowitz, referring to a few Small Business Innovation Research contracts with the military, only one of which was directly related to the shear stress sensor. Then, in 2010, IC2 landed Phase I and II SBIR contracts with Langley Research Center to work on a MEMS skin friction sensor. This led to several more NASA SBIR contracts, most of them with Langley.
“That was our first steady revenue to lead to full-time employees and eventually a commercial product,” says Horowitz, noting that the company has seen 20 to 30 percent annual growth ever since. “For us, it was a pivotal time.”
Technology Transfer
The sensor IC2 built under the 2010 contracts used the same concept as the company’s current commercial product, known as DirectShear: a micromachined silicon sensor that moves back and forth as air passes over it. A voltage is applied across two tiny pairs of parallel plate capacitors in the sensor’s surface, and their electrical capacitance varies slightly as they move, creating a change in the output voltage that is directly related to wall shear stress.
“The first ones were large, bulky, and short on performance,” says David Mills, the company’s vice president of engineering. He notes that NASA ended up investing almost $2 million in the technology over nearly 10 years, including three Phase III SBIR contracts.
McGinley, who oversaw IC2’s original NASA SBIR work, says building a sensor that works is only the first challenge. The final product also has to be resistant to swings in temperature, pressure, and humidity, as well as electronic noise. “Getting those things solved, that’s the hard problem,” she says.
The company announced the DirectShear sensor line, its first commercial product, in early 2017. The product line includes six sensor heads varying in sensitivity, resolution, and bandwidth depending on the force of the shear stress they’re designed to measure. Made for use at subsonic wind speeds—that is, slower than the speed of sound—the six versions together can sense, with high fidelity, shear stress levels from about 5,000 pascals down to 20 micropascals, the latter being about equivalent to the force of the slightest sound audible to the human ear. Shear stress forces are typically orders of magnitude lower than atmospheric and acoustic pressure levels, which is part of what makes measuring them so difficult, Horowitz says. The sensors need to measure these slight forces while remaining insensitive to pressure.
DirectShear sensors are not only the first commercial product to directly measure shear stress but also the first that can easily observe and quantify the effects of both mean flow—the overall stress air puts on the vehicle—and fluctuating turbulent air structures. The latter requires the devices to have a high bandwidth, up to 20,000 cycles per second. “Our DirectShear sensors can replace two families of technology,” Mills says.
Benefits
Shear stress accounts for about half of a vehicle’s overall drag. Understanding that drag tells a designer how a planned vehicle will perform and how far it could go on a given amount of fuel, McGinley says.
She’s been working with other NASA scientists, as well as industry and academic representatives, on a project known as the Juncture Flow Experiment, aimed at better understanding and predicting complex airflows where an aircraft’s wing meets its body. The team has been using DirectShear sensors in wind tunnel experiments with success.
In addition to improving subsonic wind tunnel testing, DirectShear sensors can also significantly improve computational fluid dynamics (CFD) software, which designers use to digitally predict a vehicle’s performance. McGinley says improving CFD technology was the main motivation behind both the Juncture Flow Experiment and Langley’s interest in IC2’s sensors. The software represents a huge cost savings over physical wind tunnel testing, but only to the extent that it’s accurate.
CFD’s predictive powers break down when confronted with novel vehicle designs or complex airflow separations. For example, McGinley says, at the outset of the Juncture Flow Experiment, each participant used its own CFD software to try to predict the nature of the airflow separation bubble that would form at a particular wing-body juncture. No two programs produced the same results.
NASA has long been both a leading pioneer and a heavy user of CFD software, although many others, from commercial airplane manufacturers to automakers, have adopted the technology.
IC2 is now in talks with government agencies, academic researchers, and space and aeronautics companies interested in buying DirectShear sensors for improved wind tunnel testing, Horowitz says. “More accurate measurement is essential to making a better-performing design and trusting that it’s going to work in the field.”
Better performance means less fuel burned, lowering both costs and emissions, as well as less noise. “Given the amount of fuel an airliner burns and how many airliners are in the air,” says Horowitz, “you can make a big difference even if you reduce drag by just a small percentage.”
He credits the SBIR program with making the DirectShear line possible. While the market for improved CFD software will be relatively large, the wind tunnel testing community is not, meaning the product’s decades of development won’t yield the sort of big, immediate payoff that venture-capital investment would have demanded. That said, he adds, “As we drive down complexity, we hope to develop a larger market outside the core science community.”
The company is preparing to bring a number of other wind tunnel testing instruments to market in the coming years, from new DirectShear products to high-frequency dynamic pressure sensors and aeroacoustic microphones, all based on the same MEMS techniques and know-how it developed to create its first product, Horowitz says. “And we used the same SBIR model of development across all of them.”
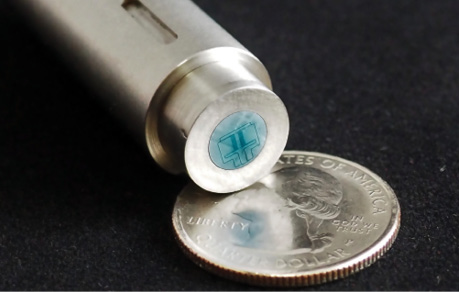
In IC2’s DirectShear sensors, a tiny, micromachined silicon sensor moves back and forth as air passes over it, changing the output voltage to indicate shear stress.
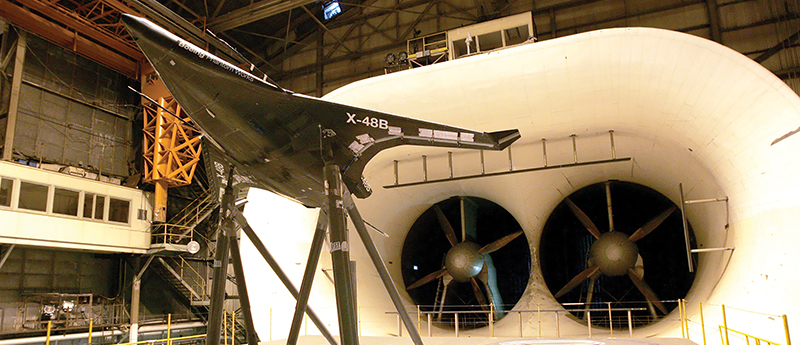
The Boeing X-48 blended wing body was the last test subject in Langley Research Center’s Full Scale Wind Tunnel, which was demolished in 2011 after 80 years of service. Langley has several wind tunnels onsite and a long, rich history of testing models and full-scale air- and spacecraft. In recent years, researchers there have been working with a brand-new wind tunnel testing capability—direct sensing of shear stress with Interdisciplinary Consulting Corporation’s (IC2) DirectShear sensors.